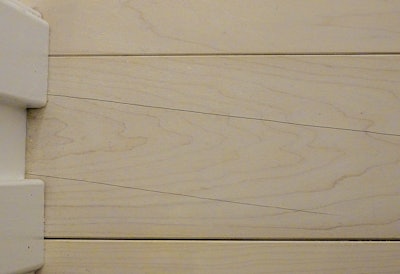
The old maxim in the wood flooring industry is that “water and wood don’t mix,” but in reality, we know that, of course, they mix whether we want them to or not. It’s managing how they mix—the moisture content of the wood—that is so important, since MC-related problems account for most wood flooring failures. Previously, we’ve taken a deep dive into the relationship between MC and board dimensions (see my article “Understand the Science of Water and Wood Floors”). Here, we will look at a key factor in wood’s MC: relative humidity. What effect does RH have on wood, exactly? It seems intuitive that RH might influence MC, but the better you understand RH, the better chance you have of avoiding problems.
Water vapor explained
To see why RH drives wood’s MC, let’s first talk about water vapor. You can see liquid water, but you cannot see water vapor. A small drop of water placed in a sealed glass jar will disappear as it evaporates, but the water is still there in the form of water vapor. A larger quantity of water placed in the bottom of the sealed jar will evaporate until the water vapor saturation pressure is reached in the upper part of the jar. At saturation, the RH is 100%. RH lower than 100% reflects a water vapor pressure that is a percentage of this maximum.
Although humidity is not directly visible, we all see and feel the effects. With high humidity, water quickly accumulates on cold drinks, doors can stick, drying is slow, mold can form, and hot days feel oppressive. It might be surprising to note that the atmosphere is only 1.5% water vapor at 100% RH and 70 degrees Fahrenheit. At the other extreme, low humidity is associated with dry skin, irritated eyes, static electricity sparks, and increased risk of infection.
What’s happening with molecules
Molecules are close together in liquid water, and the attraction between adjacent molecules keeps it together. Surface tension results from the water molecules “sticking” together. There is also a distribution of thermal (kinetic) energies, meaning some of the molecules with higher energy continually escape from the surface of the liquid into the surrounding space (evaporate). At the same time, some of the water vapor molecules impact the surface of the liquid and become part of the liquid (condense). Equilibrium is reached when the rate of evaporation is equal to the rate of condensation. The saturation pressure is reached when the liquid water and the water vapor reach equilibrium. The amount of water in the vapor can be expressed in terms of the RH; the RH is 100% at saturation.
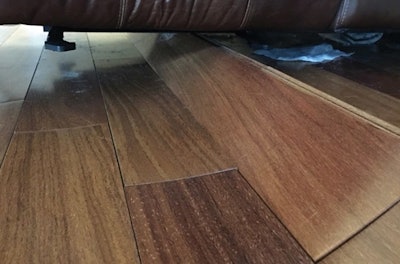
There is one more relationship that will come up again when we discuss wood: If the attraction between molecules is greater, fewer escape at the surface, and the equilibrium pressure is lower. More on balancing a change in molecular attraction with a change in equilibrium pressure soon ...
What this means for wood
Living trees depend on water for food production and to transport vital compounds along the trunk. Recently harvested lumber contains a significant amount of water in the cells. When the MC of green wood is brought down to the fiber saturation point (FSP), all the liquid water is removed from the lumens or voids within the cells. The FSP is in the MC range of 25% to 30% for many species. When wood is at equilibrium at the FSP, you expect the RH to be about 100%. At the FSP and above, the cell walls contain the maximum amount of bound water.
In constant conditions, the bound water will eventually reach equilibrium with the surrounding water vapor. This moisture level is the equilibrium moisture content, EMC. At equilibrium, the rate of water leaving the cell walls is the same as the rate of new molecules binding to cell wall material (similar to equilibrium seen in water).
However, once some water is bound to the cell walls, additional water is attracted less strongly. Cell walls with a high MC (with more bound water) attract additional water molecules less aggressively than low MC material (with less bound water). At high MC, loosely bound water molecules escape at a higher rate. If more water escapes from the cell walls, a larger supply of water vapor from the surroundings—corresponding to a higher vapor pressure, or higher RH—is needed to maintain the same EMC.
At the upper limit of the ideal MC range for flooring, wood is at an EMC of 9%. Some of the most striking wood floor failures occur when the MC of the flooring increases after it is installed. I have seen an increase in MC after installation cause flooring to lift completely off of the subfloor with both mechanically fastened and glued down floors. Dense tropical woods require extra care and can exert a significant force—enough to move an exterior door frame, for example. I even was told of mushrooms growing in a wood floor under the grand piano when moist air was allowed into the space below the floor.
At low MC, the attraction for water molecules is higher, so fewer leave the wood. Because fewer escaping molecules need to be replaced, less water vapor is needed to maintain equilibrium. Below the lower end of the ideal range, where wood has an EMC of 6%, gapping or dry cupping of engineered flooring may occur in the winter heating season. HVAC capacity mismatch can also lead to low humidity. I have seen a malfunctioning HVAC system or a wood stove heating system dry wood floor planks enough so that the tongue and groove were completely disengaged and you could see the subfloor between the floorboards.
How does temperature affect RH and EMC?
Changes in temperature have a strong effect on RH, and a less pronounced effect on EMC.
When the temperature increases 20 degrees Fahrenheit, the saturation pressure (100% RH) approximately doubles in the temperature range found in most buildings. As the temperature goes up, the rate at which water molecules escape from liquid water increases because the liquid water molecules have more thermal energy. Doubling the saturation value by increasing the temperature halves the RH. In regions where cold outside air infiltrates warm buildings, the interior RH is driven down. If the resulting MC is low enough, gaps appear in the flooring.
Conversely, cooling warm outside air from 90 degrees with a 50% RH to 70 degrees increases the RH to 100%. Liquid will form if any further cooling takes place below the dew point, and the resulting condensation causes adverse consequences to wood products in a building. In warm climates, this can occur at a boundary between the cooler air conditioned interior air and warm, humid exterior air. Without appropriate air, thermal and water barriers, elevated MC can cause floors to expand enough to buckle, or wood to decay, and mold. Complete wood floor failure is the unfortunate result in some cases. Water condensing on surfaces cooled by exterior air or by contact with the ground is another source of problems.
The rate at which bound water molecules escape from cell wall molecules also increases as the temperature goes up—again, due to the higher thermal energy. In order to maintain the same EMC, the RH needs to be increased as the temperature is increased. The other way to look at it is: The MC will decrease if the temperature goes up while the RH is constant. However, this effect is not large for the temperature range found in most building interiors.
RH determines long-term performance
Although we do not see water vapor, it has an important effect on wood. When you are developing procedures to maximize the performance of a wood floor, it starts with good manufacturing controls, then continues during distribution and storage. Proper MC at the time of installation, a correctly prepared job site, and the RH range in a building during the life of the floor determine the long-term performance of the wood floor.